Details of the bronchial tree formation remain unknown because of the difficulty in analyzing extremely small embryos. We aimed to elucidate the morphogenesis of the human embryonic bronchial tree using phase-contrast X-ray computed tomography (PXCT) images. The three-dimensional (3D) branching of the bronchial trees was reconstructed using PXCT images in a sample of embryos (between n. The images revealed that branching variants arose during the embryonic period and continued throughout life. All proximal bronchi, except the were formed by a monopodial branching mode. The 3D reconstructions of the embryonic bronchial trees provided novel insights into how bronchial trees are generated in the small embryos.
The bronchial tree of the human lung is composed of conducting and respiratory airways [1]. This organ has a highly ramified structure in the lungs. An understanding of branching morphogenesis is essential for the diagnosis and treatment of congenital anomalies. However, how such complicated branching networks are generated during development remains unclear because of the difficulty in analyzing extremely small embryos. Recently, we provided new insights into the branching tree formation in the human embryonic lung by analyzing 3D reconstructions of the human embryonic bronchial tree [2, 3].
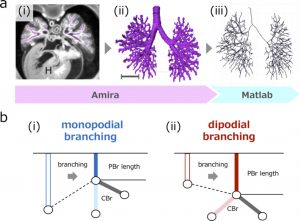
a (i) Transverse section using phase-contrast X-ray computed tomography. Heart (H). (ii) Reconstructed bronchial tree. Scale bar: 1mm. (iii) Centerline was calculated based on reconstruction. b The parent bronchus (PBr) length may shrink with monopodial branching just after generation of child bronchus (CBr) (i), but may not shrink or elongate with dipodial branching (ii).
First, we extended the morphological analysis to the end of the embryonic period [2]. The embryonic period is from the time of fertilization to 8 weeks post-fertilization. It begins with the formation of the body structures, generally described by a standardized system of 23 stages called the Carnegie stages (CS) [4]. Previous morphological studies have demonstrated the general morphogenetic processes of the human bronchial tree during the embryonic period. The primordium of the tree buds extends from the middle of the foregut at approximately CS 12 (at approximately 4 weeks after fertilization) [5]. After that, this pulmonary primordium bud continues to extend and branches out continuously to form the lobar, segmental, and more peripheral branches. The first generation of sub-segmental bronchi is complete at CS19 [6]. However, morphological changes in the trees after CS20 have not been elucidated.
Second, we analyzed how the proximal bronchus of the human lung branched off [3]. Previous studies have proposed two simple branching modes: monopodial and dipodial [7, 8]. With monopodial branching, the child branches extend from the sidewall of the parent branch. With dipodial branching, the tip of the bronchus bifurcates. Previous studies estimated the branching mode based only on visual assessments.
Thus, we aimed to describe the morphogenesis of bronchial trees during the human embryonic period. We reconstructed 3D branching trees using phase-contrast X-ray computed tomography (PXCT) images, observed the morphological changes in the trees in detail, and categorized the branching mode as monopodial and dipodial based on the bronchus length.
A total of 48 embryos between CS15 and CS23 (about 5-8 weeks after fertilization, 8-30 mm crown-rump length) [4] of the Kyoto Collection were selected [9]. Imaging data of all samples were acquired using PXCT. The system was set up at the vertical wiggler beamline (BL-14C). The PXCT imaging data provided a resolution of ≥ 18 μm/pixel [10], which enabled the non-destructive observation of intrabody structures in detail, and the highly sensitive morphometry of the embryos. The structure of the bronchial tree was reconstructed for all samples using Amira software (version 6.2.0; Visage Imaging GmbH, Berlin, Germany) (Fig. 1a). The center of the airway was observed linearly with the centerline module. The coordinates were analyzed using MATLAB v. R2018a (MathWorks, Inc., Natick, MA, USA) to calculate the generation of all branches and branch lengths.
We categorized the branching modes of the lobar, segmental, and subsegmental bronchi. After calculating each bronchus length, we categorized the branching mode of the analyzed bronchi based on whether the parent bronchus was divided after generating the analyzed bronchi (Fig.1b).
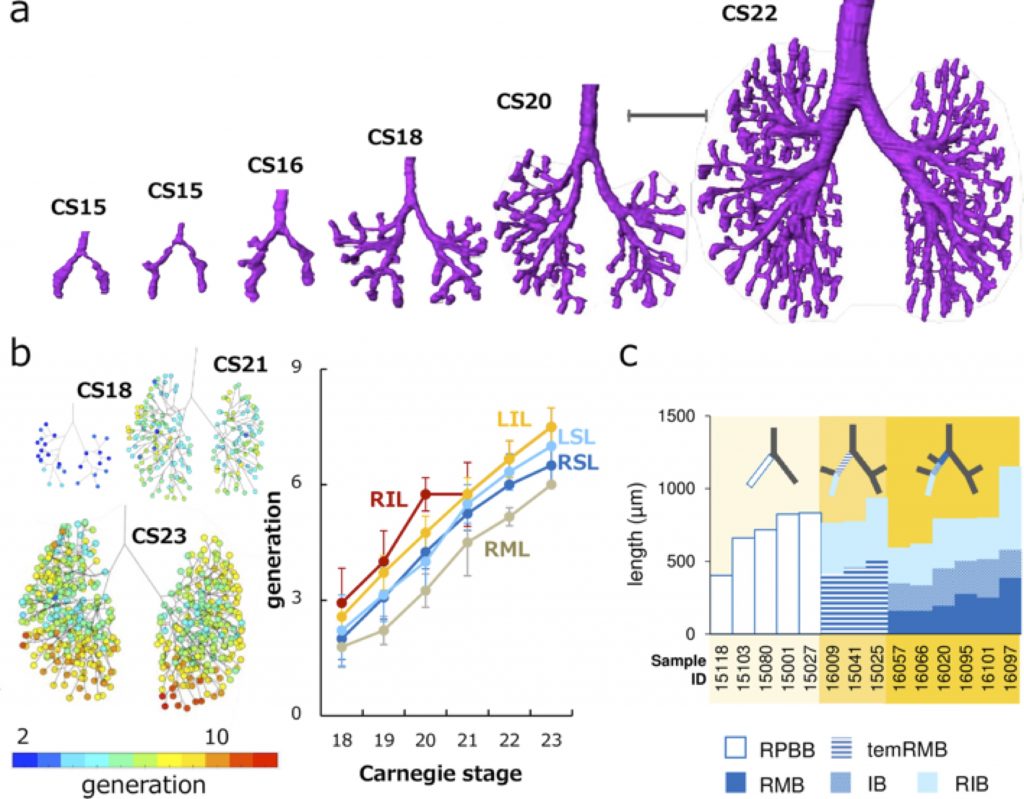
a Representative reconstructions of the bronchial tree. Scale bar: 1mm. b (left) Generation of end-branching (rainbow color) at CS18, CS21, and CS23. The colored circle indicates generations of each end-branch when the lobar bronchus was defined as 0th branch. Color bar indicates the corresponding colors. (right) Change of generation in each lobe by Carnegie stage during CS18 and CS23. LIL, left inferior lobe; LSL, left superior lobe; RIL, right inferior lobe; RML, right middle lobe; RSL, right superior lobe. c Branching mode of the right superior and middle lobar bronchi. The length changes of the right proximal bronchi are shown. Compared with the right primary bronchial bud (RPBB) length before branching, temRMB length and total length of right main bronchus (RMB) and intermediate bronchus (IB) were shorter. RIB, right inferior bronchus; temRMB, temporary RMB branch from the tracheal bifurcation to the base of the right middle lobe.
Three-dimensionally reconstructed bronchial trees revealed a timeline of morphogenesis during the embryonic period (Fig. 2a). The right superior lobar bronchus was formed after the generation of both the right middle and left superior lobar bronchi. The distribution of the end-branch generation among the five lobes was significantly different (Fig. 2b). The median branching generation value in the right middle lobe was significantly lower than that in the other four lobes. Variations found between CS20 and CS23 were all described in the human adult lung, indicating that variation in the bronchial tree may arise during the embryonic period and continue throughout life.
All lobar bronchi were formed with monopodial branching (Fig. 2c). Twenty-five bifurcations were analyzed to categorize the branching mode of the segmental and subsegmental bronchi. Of these, 22 bifurcations were categorized as monopodial branching, two bifurcations were not categorized as any branching pattern, and the only lingular bronchus that bifurcated from the left superior lobar bronchus was categorized as dipodial branching.
High-resolution imaging data of human embryonic specimens using PXCT enabled the reconstruction of the three-dimensional bronchial tree, revealing morphogenetic changes during the human embryonic period. Our novel understanding of bronchial tree development will provide a crucial resource for elucidating congenital anomalies.
Fujii, T. Takakuwa (Kyoto Univ.)
REFERENCES
- Standring, S, Gray’s Anatomy (Elsevier. 2015)
- Fujii, T. Muranaka, J. Matsubayashi, S. Yamada, A. Yoneyama and T. Takakuwa, J. Anat. 237 (2020).
- Fujii, T. Muranaka, J. Matsubayashi, S. Yamada, A. Yoneyama and T. Takakuwa, PLoS ONE 16, 1 (2021)
- O’Rahilly and F. Müller, Developmental stages in human embryos: including a revision of Streeter’s” horizons” and a survey of the Carnegie Collection, (Carnegie Institution of Washington Publishing. 1987).
- Streeter, G.L., Contributions to Embryology Carnegie Institution of Washington, 31 (1945)
- Wells, L. and Boyden, E. American Journal of Anatomy, 95 (1954)
- Palmer, D. M. Ohio Journal of Science, 36 (1936).
- Metzger RJ, Klein OD, Martin GR and Krasnow MA, Nature. 453, 7196 (2008).
- Nishimura, K. Takano, T. Tanimura and M. Yasuda, Teratology, 1 (1968).
- Yoneyama, S. Yamada and T. Takeda, “Fine Biomedical Imaging Using X-Ray Phase-Sensitive Technique” in Advanced Biomedical Engineering, edited by G. D. Gargiulo and A. McEwan, 107-128. InTech Publishinng 2011.